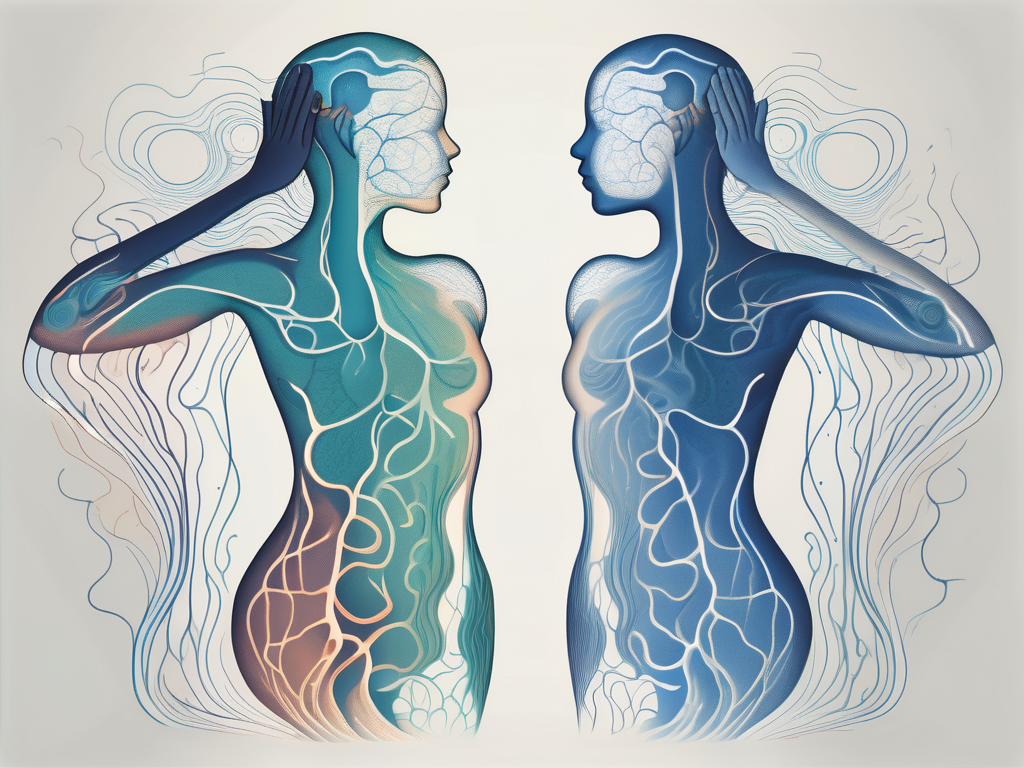
Rivers Within: Exploring the Fluid Dynamics of Human Physiology
The human body is a complex and remarkable machine, and one of the key principles that govern its functioning is fluid dynamics. Our bodies rely on the movement and interaction of various fluids, such as blood, air, and water, to carry out vital processes. In this article, we will explore the fascinating world of fluid dynamics within the human body, examining how different fluids flow through various systems and organs, and how these dynamics have evolved to optimize their function.
Blood, Air and Water - The Fluids of Biology
When we think of fluid dynamics, the first thing that may come to mind is the movement of water in a river or the flow of air around an airplane wing. While these examples are certainly relevant to the field of fluid dynamics, it is important to note that biology presents its own unique set of challenges and considerations.
The human body is a living system, constantly adapting and responding to changing conditions. As a result, fluid dynamics within the body are complex and highly regulated. Three primary fluids - blood, air, and water - play crucial roles in maintaining our health and well-being. Let's delve into each of these fluids and explore how they flow within the body.
Blood: The Lifeline of the Body
Blood, often referred to as the lifeline of the body, is a remarkable fluid that circulates through a vast network of blood vessels, delivering essential nutrients and oxygen to every cell and removing waste products. It consists of solids and liquids in almost equal proportions. The solid part of your blood includes red blood cells, white blood cells, and platelets. The liquid part is called plasma and is made up of water, salts, and protein.
Within the circulatory system, blood flows through arteries, veins, and capillaries, propelled by the rhythmic contractions of the heart. This continuous flow ensures that oxygen-rich blood reaches all organs and tissues, providing them with the necessary resources to carry out their functions.
However, blood flow is not uniform throughout the body. In areas of high metabolic demand, such as the brain and muscles during exercise, blood flow increases to meet the increased oxygen and nutrient requirements. This regulation of blood flow is crucial for maintaining the body's equilibrium and ensuring proper functioning.
Air: The Breath of Life
Air, another vital fluid in biology, is essential for the process of respiration. Through the intricate network of airways in our respiratory system, air enters our lungs, where oxygen is exchanged for carbon dioxide, a waste product of cellular metabolism.
The flow of air within the respiratory system is a dynamic process, driven by the expansion and contraction of the chest cavity. As we inhale, the diaphragm and intercostal muscles contract, creating a negative pressure that draws air into the lungs. Upon exhalation, these muscles relax, allowing for the expulsion of carbon dioxide-rich air.
Furthermore, the respiratory system is equipped with specialized structures, such as cilia and mucus, which help to filter and humidify the air we breathe. This ensures that the air reaching our lungs is clean and at the right temperature and humidity for optimal gas exchange.
Water: The Elixir of Life
Water, often referred to as the elixir of life, is a fundamental fluid that plays a vital role in numerous biological processes. It serves as a solvent for essential nutrients and waste products, aids in temperature regulation, and provides structural support to cells and tissues.
Within the body, water is distributed in various compartments, including intracellular and extracellular spaces. It moves between these compartments through osmosis, a process driven by differences in solute concentration. This movement of water ensures that cells maintain their proper shape and function.
Additionally, water is involved in digestion, as it helps break down food particles and facilitates the absorption of nutrients in the gastrointestinal tract. It also acts as a lubricant in joints, allowing for smooth movement, and helps regulate body temperature through processes like sweating and evaporation.
Air Flow in the Respiratory System
One of the most essential functions of the human body is respiration, or the exchange of gases. The lungs, situated within the thoracic cavity, are the key organ responsible for this process. The flow of air in the pulmonary system is guided by a series of intricate structures, designed to maximize the efficiency of oxygen uptake and carbon dioxide removal.
The human respiratory system is an elegant example of fluid dynamics in action. With every inhalation and exhalation, a complex series of pressure changes facilitates the flow of air into and out of the lungs. The principles of fluid mechanics govern this airflow, similar to how aerodynamics describes the motion of air around an automobile.
When we inhale, air enters through the nasal passages or the mouth and travels down the trachea, branching into smaller tubes called bronchi and bronchioles. These airways eventually lead to millions of tiny air sacs called alveoli. It is here, within the alveoli, that the exchange of oxygen and carbon dioxide takes place, facilitated by the thin walls of the pulmonary capillaries. As a result, an intricate balance of fluid dynamics is established within the lungs, ensuring that oxygen is efficiently transported into the bloodstream and carbon dioxide is effectively eliminated.
During inhalation, the diaphragm muscle contracts and the rib cage expands, increasing the volume of the thoracic cavity. This change in volume decreases the air pressure inside the lungs relative to the atmospheric pressure, causing air to flow down the pressure gradient through the airways and into the lungs, following the same laws that describe airflow through a pipe or duct. As the air reaches the alveoli, the tiny sacs where gas exchange occurs, the geometries become increasing complex, giving rise to diffusive mixing processes well-modeled by viscous flow equations. During exhalation, the process reverses as the diaphragm and intercostal muscles relax, decreasing lung volume and expelling air back out. Computational fluid dynamics techniques help researchers study and optimize these intricately coupled pressure-driven and shear-driven airflows.
Blood Flow in the Pulmonary and Vascular Systems
Blood, the life-sustaining fluid of the body, transports oxygen, nutrients, hormones, and waste products to and from various tissues and organs. This complex network of vessels, collectively known as the vascular system, ensures the efficient flow of blood throughout the body.
The main components of the vascular system are arteries, which carry oxygenated blood away from the heart, and veins, which transport deoxygenated blood back to the heart. These vessels are connected by a vast network of smaller blood vessels called capillaries, where the exchange of substances between blood and tissues occurs.
The flow of blood in the vascular system is intricately regulated by diverse factors, such as blood pressure, vessel diameter, and the contraction of smooth muscles within the vessel walls. By adjusting the diameter of blood vessels, the body can control the distribution of blood to different organs and tissues based on their metabolic demands.
Furthermore, our bodies have cleverly evolved to overcome the effects of gravity on blood flow. Valves within veins prevent the backflow of blood, and muscular contractions assist in propelling blood back towards the heart. These mechanisms ensure efficient circulation, allowing for the delivery of essential nutrients and the removal of waste products throughout the body.
The vascular system is a remarkably complex circulatory network that relies heavily on the principles of fluid dynamics to function properly. The heart acts as a muscular pump that generates the driving force to propel blood through the body's vast system of blood vessels. As the heart contracts, it creates pressure gradients that cause blood to flow from regions of high pressure to low pressure, much like water flowing through a hose.
The flow patterns of blood exhibit many characteristics that can be described and analyzed using fluid dynamics concepts. Blood behaves as a non-Newtonian fluid, meaning its viscous properties change based on the shear forces acting upon it. In larger vessels like arteries, blood flow is streamlined and can be modeled using the Navier-Stokes equations that govern viscous fluid flow. However, in smaller vessels and capillaries, the particle nature of blood becomes more pronounced, requiring different models that account for complex factors like the interactions between plasma and formed elements like red blood cells. Understanding these fluid dynamics is crucial for investigating cardiovascular disorders, optimizing surgical techniques, and developing cardiovascular implants that promote ideal blood flow patterns.
Flow in the Digestive System
The digestive system relies heavily on the physics of fluid flow to transport and process the foods and liquids we consume. When we consume food and drink, the initial processing begins in the mouth, where water-based saliva becomes mixed with the ingested material. From the moment we swallow, the principles of fluid mechanics come into play as the bolus of food travels down the esophagus aided by coordinated contractions and relaxations of the muscle lining. This mixture then travels to the stomach, where it is further broken down by gastric secretions. Eventually, the partially digested material passes into the small intestine, where the majority of nutrient absorption takes place.
Once in the stomach, the food is broken down into a semiliquid state called chyme, which exhibits complex non-Newtonian fluid behavior as it is agitated and churned by contractions of the stomach walls. The flow properties and mixing dynamics of this stomach chyme are crucial for proper digestion and nutrient absorption. As the chyme is released into the small intestine, peristaltic motion creates precisely timed pressure pulses that facilitate flow, while optimized fluid dynamics assists in maximizing the absorption area along the villi lining.
Fluid flow also plays a vital role in other systems like the renal system, where the volumetric regulation and pressure-driven filtration processes in the kidneys govern blood filtration and urine production. Even in synovial joints, the unique flow characteristics of synovial fluid help provide lubrication and load transfer between bones. Modeling these biologic flows using fluid dynamics enhances our understanding of healthy function as well as disease states.
Within both systems water acts as a solvent, aiding in the breakdown and absorption of nutrients. It also helps maintain the consistency of the intestinal contents, ensuring smooth movement and preventing constipation.
Moreover, the flow of water within the digestive system is intricately linked to the regulation of electrolytes and osmotic pressures. This delicate balance allows for the controlled absorption and secretion of substances, ensuring that water is effectively absorbed into the bloodstream while waste products are efficiently eliminated.
How Body Systems and Organs Have Evolved to be Flow Optimized
As we have explored the different fluid dynamics within the human body, it becomes evident that the various systems and organs have evolved in remarkable ways to optimize flow. Evolution has fine-tuned the structures and mechanisms involved in fluid dynamics, ensuring the efficient functioning of our bodies and the maintenance of homeostasis.
The human heart exemplifies how evolution has sculpted organs for optimal fluid dynamics. The heart's four chambers are arranged in a circular loop, allowing blood to flow through the pulmonary and systemic circulations efficiently. The ventricles feature curving outer walls and cone-shaped contracting motions that create a spiral, vortex flow pattern to prevent energy losses and promote smooth ejection of blood. Additionally, the aortic valve's three leaflets open fully during systole to minimize obstructions, while the semilunar shape of the valve pockets prevents backflow during diastole.
The lungs have also undergone exquisite evolutionary refinement for air flow and gas exchange optimization. The bronchial tree's asymmetric branching pattern provides an efficient airway geometry that reduces airflow resistance compared to a symmetrical bifurcating pattern, ensuring that every cell in the body is within a short distance of a nutrient-rich blood supply. Additionally, the space-filling fractal nature of the airways progressively increases the cross-sectional area to decelerate flow velocity as the airways subdivide further. At the alveolar level, the lung's enormous surface area facilitates rapid diffusion for pulmonary gas exchange.
Even single-celled organisms like the paramecium exhibit flow-optimized adaptations. These protists have a concave-shaped body and thousands of tiny hair-like motile appendages called cilia covering their surface. Through coordinated beating of the cilia, paramecia are able to create hydrodynamic waves that propel them through fluid and external flows in optimized swimming and feeding patterns. The fluid dynamics generated by the cilia are precisely tuned for their size and environmental conditions.
The efficiency of water and nutrient absorption in the digestive system is boosted by the presence of microvilli, tiny finger-like projections that increase the surface area available for absorption.
These adaptations, among many others, highlight the remarkable interplay between form and function in the human body.
Cutting-edge Applications of Fluid Dynamics in Biomedical Engineering
Fluid dynamics plays an increasingly important role in advanced medical diagnostics and treatments. For example, computational fluid dynamics (CFD) simulations are used to optimize the design of medical imaging devices like CT and MRI scanners to control the flow of ionizing particles, contrast agents, and cooling fluids. CFD also aids in developing improved ultrasound probes and transducer geometries to generate ideal acoustic beam profiles for enhanced image quality.
In the treatment realm, modeling fluid behavior is crucial for novel drug delivery methods like nanoparticles and aerosol administration of pharmaceuticals. Understanding the fluid dynamics of how particles disperse and deposit in the respiratory tract allows for more effective and targeted pulmonary drug delivery. Similarly, fluid simulations guide the design of microneedle array patches to control interstitial fluid dynamics during transdermal drug administration.
Fluid dynamics has become indispensable in biomedical engineering of implantable devices and artificial organs. For cardiovascular implants like stents, valves and ventricular assist devices, CFD optimizes geometries to promote ideal blood flow patterns that prevent thrombosis risk, hemolysis, and flow-induced stresses. For renal dialysis, accurate fluid models of the flow dynamics in hollow fiber membrane bundles enable the development of more efficient blood filtration systems.
At the cutting edge, some researchers are even leveraging fluid dynamics for novel cancer therapies. Acoustic streaming induced by an ultrasonic beam can create microscale fluid flows that enhance penetration and binding of anti-cancer drugs into tumor cells. Other approaches use fluid forces generated by microfluidics to mechanically disrupt cellular structures and membranes. As our understanding of biologic fluid behavior advances, the applications of fluid dynamics in medicine will continue to accelerate.
Fluid-Structure Interaction (FSI) Simulation of an Artificial Heart Valve
Computational Fluid Dynamics (CFD) Simulation of a Metered-Dose Inhaler
Conclusion
In conclusion, fluid dynamics within the human body are a fascinating and essential aspect of our physiological processes. The flow of blood, air, and water is intricately regulated to maintain homeostasis and ensure the optimal functioning of our organs and systems. Understanding these fluid dynamics not only enhances our knowledge of human biology but also provides insights into the evolution of life itself. So, the next time you take a breath, feel your heartbeat, or quench your thirst, appreciate the remarkable fluid dynamics at work within your body.