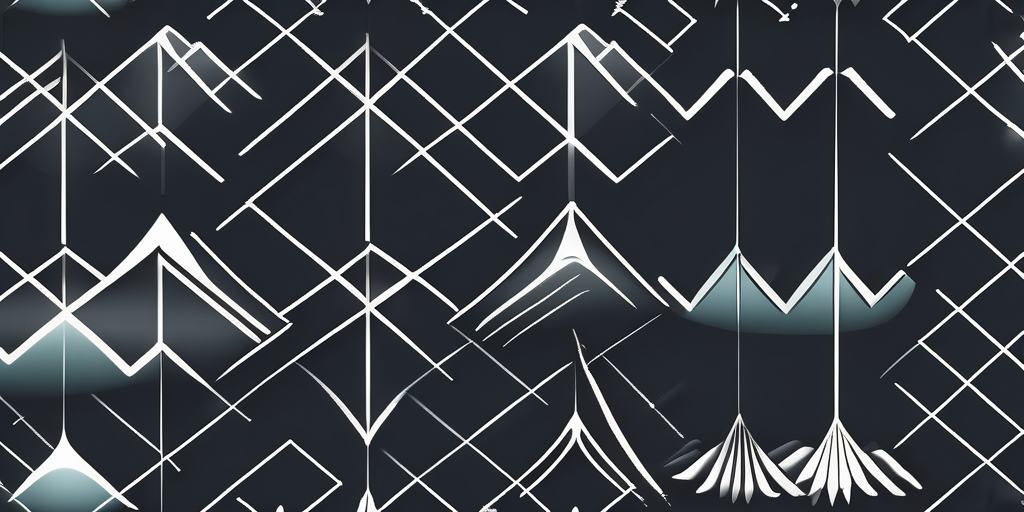
An Introduction to Applied Aerodynamics
What is Aerodynamics?
Aerodynamics is the branch of physics that deals with the study of how air moves around objects in motion, particularly aircraft. It plays a crucial role in the design and performance of airplanes, helicopters, and even cars. By understanding the principles of aerodynamics, engineers can optimize the shape and structure of vehicles, making them more efficient and safer to operate.
The Continuum Assumption
One of the fundamental assumptions in aerodynamics is the continuum assumption, which states that air is a continuous fluid with uniform properties. This assumption allows engineers to simplify complex fluid dynamics problems by treating air as a continuous substance rather than as individual molecules. By adopting the continuum assumption, scientists can use classical physics principles, such as Newton's laws of motion, to analyze and predict the behavior of air around flying objects.
When considering the continuum assumption, it is important to understand the concept of fluid continuity. This principle states that within a fluid, such as air, the properties at any given point are smoothly distributed and can be described by continuous functions. This means that the velocity, pressure, and temperature of the air can vary continuously as one moves through it.
By assuming air as a continuous fluid, engineers can apply mathematical models, such as the Navier-Stokes equations, to analyze the flow of air around aircraft, rockets, and other flying objects. These equations, derived from the principles of fluid mechanics, describe the conservation of mass, momentum, and energy within a fluid. They allow engineers to calculate important parameters, such as lift, drag, and thrust, which are crucial for designing efficient and safe aircraft.
However, it's important to note that the continuum assumption may not hold true in certain extreme conditions, such as at very high altitudes or in the presence of rarefied gases. At high altitudes, the density of air decreases significantly, and the mean free path of air molecules becomes comparable to the characteristic length scales of the flow. In these cases, the behavior of air might deviate from the predictions based on the continuum assumption.
When the continuum assumption breaks down, engineers and scientists must turn to specialized mathematical models and experimental techniques to accurately describe the flow. For example, in rarefied gas dynamics, the behavior of individual molecules becomes important, and statistical methods, such as the Boltzmann equation, are used to describe the flow at the molecular level. These models take into account the collisions and interactions between individual molecules to predict the macroscopic behavior of the gas.
Experimental techniques, such as wind tunnel testing and computational fluid dynamics simulations, are also used to study flows where the continuum assumption is not valid. These methods allow engineers to visualize and analyze the complex flow patterns that arise in these extreme conditions. By combining theoretical models with experimental data, engineers can gain a comprehensive understanding of the flow behavior and make informed design decisions.
In conclusion, while the continuum assumption is a powerful tool for simplifying aerodynamic analysis, it is important to recognize its limitations. In certain extreme conditions, such as at high altitudes or in rarefied gases, the behavior of air may deviate from the predictions based on the continuum assumption. By employing specialized mathematical models and experimental techniques, engineers can overcome these challenges and continue to advance our understanding of fluid dynamics in a wide range of applications.
The Four Forces of Flight
In aerodynamics, there are four primary forces that act on an aircraft during flight: lift, weight, thrust, and drag. These forces play a crucial role in determining the behavior and stability of an aircraft in the air.
Lift is the force that opposes gravity and allows an aircraft to stay aloft. It is generated by the interaction between the wings and the airflow passing over them. By carefully shaping the wings and controlling the angle of attack, engineers can maximize the lift produced by an aircraft, enabling it to achieve and maintain level flight.
Weight, on the other hand, is the force exerted by gravity on an aircraft. It acts vertically downward and must be counteracted by lift to keep the aircraft airborne. By carefully managing the weight distribution and structural integrity of an aircraft, engineers can ensure that it remains balanced and stable during flight.
Thrust is the force that propels an aircraft forward. It is typically generated by engines or propellers and is responsible for overcoming the drag force. By producing sufficient thrust, an aircraft can achieve and maintain the desired speed and altitude, allowing it to travel efficiently through the air.
Drag is the force that resists the motion of an aircraft through the air. It is caused by the friction between the aircraft's surface and the air molecules surrounding it. The design and aerodynamic features of an aircraft, such as its streamlined shape and smooth surfaces, play a crucial role in minimizing drag, thereby reducing fuel consumption and improving overall performance.
Let's dive deeper into each of these forces to understand their impact on flight.
Lift: Lift is created by the difference in air pressure between the upper and lower surfaces of an aircraft's wings. As the aircraft moves through the air, the shape of the wings and the angle at which they meet the airflow determine the amount of lift generated. By increasing the angle of attack, pilots can increase lift, allowing the aircraft to climb. Conversely, decreasing the angle of attack reduces lift, facilitating descent. The careful management of lift is crucial for maintaining altitude and controlling the aircraft's vertical movements.
Weight: Weight is the force exerted by gravity on an aircraft and is directly proportional to its mass. To keep an aircraft in equilibrium, lift must equal weight. Engineers carefully consider weight distribution during the design phase to ensure that the aircraft remains balanced. Additionally, structural integrity is crucial to withstand the forces exerted on the aircraft during flight. By managing weight and balance, pilots can ensure safe and stable flight conditions.
Thrust: Thrust is the force that propels an aircraft forward. It is generated by engines or propellers, which convert fuel into mechanical energy. The amount of thrust produced determines the aircraft's speed and acceleration. Pilots control thrust by adjusting the throttle or power settings of the engines. During takeoff, pilots apply maximum thrust to achieve the necessary speed for lift-off. Once in the air, they adjust thrust to maintain the desired speed and altitude. Efficient thrust management is crucial for achieving optimal flight performance and fuel efficiency.
Drag: Drag is the resistance encountered by an aircraft as it moves through the air. It is caused by various factors, including the shape of the aircraft, surface roughness, and air viscosity. Engineers employ various techniques to minimize drag, such as designing streamlined shapes, smoothing surfaces, and reducing protrusions. By reducing drag, aircraft can achieve higher speeds and consume less fuel. Pilots also play a role in managing drag by adjusting the aircraft's configuration, such as retracting landing gear and adjusting flaps, to optimize aerodynamic efficiency.
Understanding the four forces of flight is essential for pilots, engineers, and aviation enthusiasts alike. By comprehending how lift, weight, thrust, and drag interact, we can appreciate the complexities of flight and the remarkable achievements of aviation technology. Whether you're soaring through the sky or marveling at an aircraft from the ground, these forces are at the core of every flight.
Subsonic, Transonic, Supersonic and Hypersonic Flows
Aerodynamic flows can be classified into different regimes based on the speed of the airflow relative to the speed of sound. How is subsonic flow defined? In subsonic flow, the velocity of the airflow is lower than the speed of sound. This is the most common regime for most everyday objects, including cars and commercial airplanes.
Transonic flow occurs when the airflow is close to the speed of sound. It is a challenging regime for aerodynamic design and requires specific techniques to manage the shockwaves and flow separation that occur at these speeds.
Supersonic flow happens when the airflow is faster than the speed of sound. In this regime, shockwaves are generated and can have a significant impact on the aerodynamic performance of an aircraft.
Hypersonic flow, on the other hand, is characterized by velocities much higher than the speed of sound. In this regime, the airflow properties drastically change due to the intense heating and compression effects caused by the high speed. Hypersonic flows are of particular interest in the design and analysis of space vehicles and high-speed aircraft.
Boundary Layers and Turbulence
When air flows over a surface, such as an aircraft wing, it creates a thin layer called the boundary layer. The boundary layer can be divided into two regions: the laminar boundary layer and the turbulent boundary layer.
In the laminar boundary layer, the air flows smoothly and in a well-ordered manner. However, as the airflow continues along the surface, it can become unstable and transition into a turbulent flow. Turbulent flow is characterized by chaotic and unpredictable movement of the fluid particles, creating vortices and eddies.
Turbulent flows have higher energy and can significantly impact the aerodynamic performance of an aircraft. Engineers need to consider and manage turbulence, using specialized techniques such as boundary layer control and turbulence models, to ensure optimal performance and stability.
Conclusions
In conclusion, aerodynamics is a complex field that encompasses the study of airflow around objects in motion. By understanding the continuum assumption, the four forces of flight, different flow regimes, and the impact of boundary layers and turbulence, engineers can design and optimize aircraft for safe and efficient operation. Applying this knowledge, they continue to advance the field of aerodynamics, pushing the boundaries of flight and enabling new innovations in aviation technology.