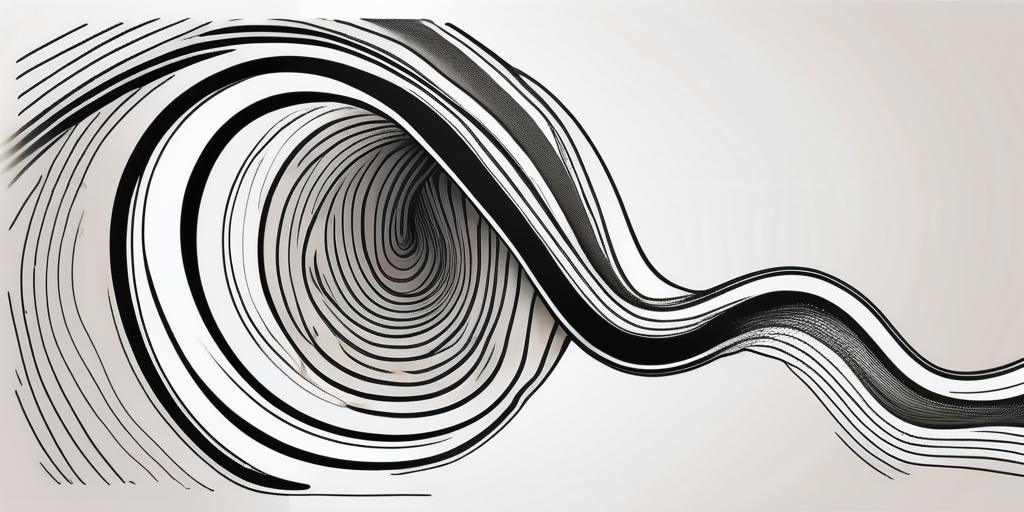
An Introduction to Laminar Flow
What is Laminar Flow?
Laminar flow is a fundamental concept in fluid dynamics that describes the smooth and ordered movement of a fluid. It occurs when a fluid flows in parallel layers with little to no mixing between adjacent layers. In laminar flow, the fluid particles move in a steady and predictable manner, creating a well-defined stream of flow.
Relationship with Reynolds' Number
One important factor that governs the nature of flow is the Reynolds' number. This dimensionless quantity is a key parameter used to determine whether flow is laminar or turbulent. Reynolds' number is calculated by dividing the product of the fluid velocity, characteristic length, and fluid density by the fluid viscosity.
For flow to be classified as laminar, the Reynolds' number must be below a critical value, typically around 2,000. At values below this threshold, the fluid behaves in a smooth and ordered manner, with minimal mixing and disturbances. However, as the Reynolds' number increases beyond this critical value, the flow transitions into a turbulent state, characterized by chaotic and unpredictable motion.
The concept of Reynolds' number was first introduced by the Irish engineer Osborne Reynolds in the late 19th century. Reynolds was conducting experiments to understand the behavior of fluid flow in pipes and channels. He observed that the nature of flow depended not only on the fluid properties but also on the size of the system and the velocity of the fluid.
Reynolds' number provides a way to compare different flow situations and predict the type of flow that will occur. It is widely used in various fields, including engineering, physics, and biology. For example, in engineering, the Reynolds' number is used to design efficient and safe pipelines, determine the performance of aircraft wings, and optimize the flow of fluids in heat exchangers.
In fluid dynamics, the transition from laminar to turbulent flow is a subject of great interest and complexity. It is influenced by various factors, such as the geometry of the system, surface roughness, and the presence of obstacles. Reynolds' number serves as a valuable tool to understand and analyze this transition.
When the Reynolds' number is below the critical value, the flow is predominantly laminar. In this regime, the fluid particles move in smooth layers, parallel to each other. The motion is highly ordered, and there is little mixing between adjacent layers. This type of flow is often observed in low-velocity situations, such as the flow of viscous liquids through narrow tubes.
On the other hand, when the Reynolds' number exceeds the critical value, the flow becomes turbulent. In turbulent flow, the fluid particles move in a chaotic and irregular manner. Vortices, eddies, and swirls are formed, leading to enhanced mixing and increased fluid resistance. Turbulent flow is commonly encountered in high-velocity situations, such as the flow of air around a moving vehicle or the flow of water in rivers.
Understanding the transition from laminar to turbulent flow is of great practical importance. Turbulent flow can have significant effects on the performance and efficiency of various systems. It can increase drag, reduce heat transfer rates, and cause structural vibrations. By studying the relationship between Reynolds' number and flow behavior, engineers and scientists can design better systems and optimize their performance.
Real World Examples
Laminar flow can be observed in various natural and man-made systems. One common example is the flow of blood in blood vessels. When the flow is smooth and steady, it allows for efficient transport of oxygen and nutrients to the body's tissues. This is crucial for maintaining the overall health and well-being of an individual. The laminar flow in blood vessels ensures that the blood cells can easily navigate through the narrow passages without any disruptions or blockages. It also helps in preventing the formation of blood clots, which can have serious health consequences.
Similarly, the flow of air in the respiratory system during normal breathing is predominantly laminar. This ensures that the oxygen we inhale is efficiently transported to the lungs, where it can be absorbed into the bloodstream. The laminar flow in the respiratory system also helps in filtering out any impurities or particles present in the air, ensuring that we breathe in clean and fresh air.
In engineering applications, laminar flow is utilized in industries such as aerospace and automotive. For instance, in aircraft design, laminar flow wings are employed to reduce drag and increase fuel efficiency. The smooth and ordered flow over these wings minimizes turbulence and improves aerodynamic performance. This not only allows the aircraft to fly more efficiently but also reduces the noise generated during flight, making it more environmentally friendly.
Additionally, laminar flow is critical in heat exchangers, where it facilitates efficient heat transfer by promoting uniform fluid contact with heat transfer surfaces. Heat exchangers are widely used in various industries, including power plants, chemical processing, and refrigeration systems. The laminar flow ensures that the heat is effectively transferred from one fluid to another, maximizing the overall efficiency of the system. This is particularly important in energy-intensive processes, where even a small improvement in heat transfer can result in significant energy savings.
Furthermore, laminar flow is also utilized in the pharmaceutical industry during the manufacturing of drugs. The controlled laminar flow environment in cleanrooms helps in preventing contamination and maintaining the sterility of the production process. This is crucial for ensuring the safety and efficacy of the medications being produced, as any contamination can have serious consequences for the patients consuming these drugs.
In conclusion, laminar flow has a wide range of applications in both natural and man-made systems. From the efficient transport of oxygen in our bodies to the design of fuel-efficient aircraft, laminar flow plays a crucial role in various industries and processes. Its ability to minimize turbulence and promote uniform fluid contact makes it an essential concept in fluid dynamics and engineering. Understanding and harnessing the power of laminar flow has led to numerous advancements and innovations, improving efficiency, performance, and safety in various fields.
The Transition to Turbulence
While laminar flow is desirable in many situations, it is susceptible to transitioning into turbulence under certain conditions. This transition is influenced by factors such as the flow velocity, flow disturbances, and surface roughness.
As the flow velocity increases, the likelihood of turbulence occurring also rises. This can be observed in situations where a fluid encounters obstacles or changes in direction, causing disruptions to the smooth flow. Additionally, any disturbances in the flow, such as an uneven surface or local pressure variations, can trigger the transition to turbulence.
Understanding the transition to turbulence is crucial in various industries, as it can have significant impacts on system performance and efficiency. Consequently, extensive research and computational models have been developed to investigate and predict the transition process, allowing engineers to design systems that optimize laminar flow and mitigate the effects of turbulence.
Conclusions
In conclusion, laminar flow is a fundamental concept in fluid dynamics that describes the smooth and ordered movement of a fluid. Its occurrence is governed by the Reynolds' number, with flow being classified as laminar below a critical value. Real-world examples of laminar flow can be found in biological systems and engineering applications. However, laminar flow is not without its limitations, as it is susceptible to transitioning into turbulence under certain conditions. Understanding the transition process is essential for optimizing system design and performance.