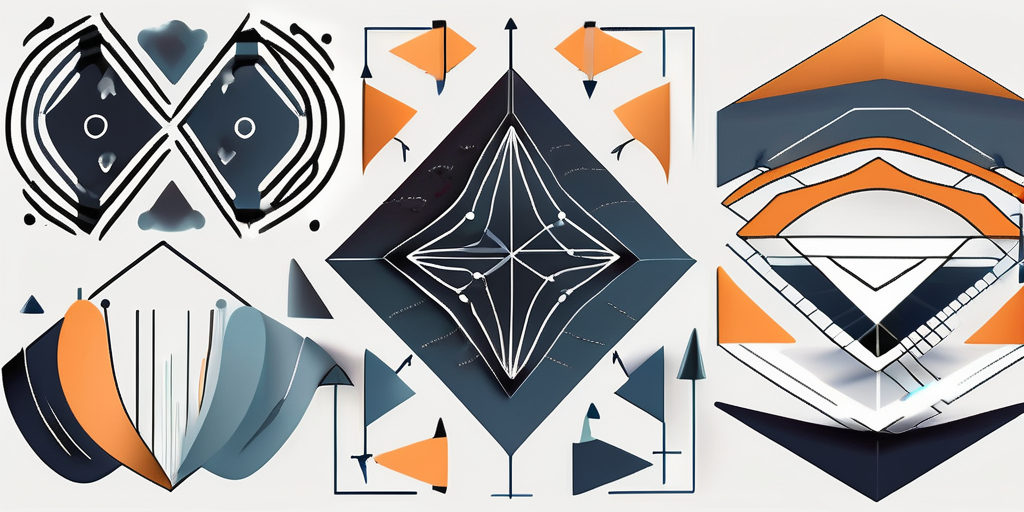
What are Common Boundary Conditions in CFD?
Common Boundary Conditions Used in Real World CFD Applications
Computational Fluid Dynamics (CFD) is a powerful tool used to solve fluid flow problems in various industries, such as aerospace, automotive, and environmental engineering. When performing CFD simulations, it is crucial to define appropriate boundary conditions to accurately model the real-world phenomenon being studied. In this article, we will explore the most commonly used boundary conditions in real-world CFD applications.
The Mass Flow or Velocity Inlet Condition
The Mass Flow or Velocity Inlet Condition is widely used when the flow rate or velocity at the inlet is known and needs to be prescribed. In this boundary condition, the flow properties, such as mass flow rate, velocity, and temperature, are specified at the inlet plane. This condition is often applied in scenarios where fluid enters an enclosed or open domain, such as in aerodynamics simulations or HVAC system analysis.
By enforcing the Mass Flow or Velocity Inlet Condition, CFD solvers can accurately simulate the flow behavior at the inlet. This allows engineers to study phenomena such as flow separation, turbulence, and pressure drop. In practical applications, this condition is utilized for designing more efficient and optimized systems by analyzing the fluid flow characteristics at the inlet.
When considering the Mass Flow or Velocity Inlet Condition, it is crucial to understand the underlying principles that govern fluid dynamics. The flow rate, which is the amount of fluid passing through a given area per unit time, plays a significant role in determining the behavior of the fluid. By specifying the mass flow rate or velocity at the inlet, engineers can control and manipulate the flow characteristics to achieve desired outcomes.
In aerodynamics simulations, the Mass Flow or Velocity Inlet Condition is particularly important. It allows engineers to accurately model the behavior of air entering an aircraft's intake or a wind tunnel. By prescribing the flow properties at the inlet, the simulation can capture the complex interactions between the air and the aircraft's surfaces, providing valuable insights into lift, drag, and other aerodynamic forces.
In HVAC system analysis, the Mass Flow or Velocity Inlet Condition is essential for designing efficient heating, ventilation, and air conditioning systems. By specifying the flow rate or velocity at the inlet, engineers can optimize the system's performance, ensuring proper air distribution and temperature control. This condition enables the simulation of various scenarios, such as different air supply velocities or multiple inlets, allowing engineers to evaluate the system's behavior under different operating conditions.
Furthermore, the Mass Flow or Velocity Inlet Condition is not limited to fluid dynamics simulations. It is also applicable in other fields, such as chemical engineering and environmental science. In chemical engineering, this condition is used to model the flow of reactants or fluids in industrial processes, enabling engineers to optimize reaction rates and product yields. In environmental science, it is employed to study the transport and dispersion of pollutants in rivers, lakes, or the atmosphere, aiding in the assessment of environmental impacts and the development of mitigation strategies.
Overall, the Mass Flow or Velocity Inlet Condition is a versatile and powerful tool in computational fluid dynamics. By prescribing the flow properties at the inlet, engineers can accurately simulate and analyze fluid flow behavior in various applications. This allows for the optimization of systems, the understanding of complex phenomena, and the development of innovative solutions across a wide range of industries.
The Pressure Outlet Condition
The Pressure Outlet Condition is a fundamental concept in computational fluid dynamics (CFD) simulations. It is used when the pressure at a specific boundary needs to be defined, allowing engineers to accurately analyze and predict fluid flow behavior in various industrial applications.
When implementing the Pressure Outlet Condition, it is commonly applied at the outlet of the computational domain. This boundary condition enables engineers to compute flow properties based on the specified pressure, providing valuable insights into system performance.
In real-world CFD simulations, evaluating pressure drops across specific components or systems is often essential. By utilizing the Pressure Outlet Condition, engineers can assess the efficiency and effectiveness of various industrial processes. For example, in exhaust systems, understanding the pressure drop can help optimize engine performance and reduce emissions.
Furthermore, the Pressure Outlet Condition allows engineers to study flow characteristics such as backflow, recirculation zones, and pressure recovery. These factors play a crucial role in ensuring the safe and efficient operation of industrial systems. For instance, in pipeline networks, analyzing flow behavior using the Pressure Outlet Condition helps identify potential areas of turbulence or pressure loss, enabling engineers to optimize the network's design and minimize energy consumption.
Cooling systems are another area where the Pressure Outlet Condition proves invaluable. By studying flow characteristics and pressure recovery, engineers can ensure efficient heat dissipation and prevent overheating in critical components. This is particularly important in industries such as automotive manufacturing, where cooling systems are essential for maintaining optimal operating temperatures.
In summary, the Pressure Outlet Condition is a versatile boundary condition in CFD simulations. Its implementation allows engineers to define pressure at specific boundaries, enabling accurate analysis of flow behavior, pressure drops, and other critical factors. By utilizing this condition, engineers can optimize system performance, ensure safe operations, and enhance the efficiency of various industrial applications.
The Wall Condition
The Wall Condition pertains to boundaries that represent solid surfaces, where no fluid can penetrate. In CFD simulations, walls are typically defined with no-slip conditions, meaning that the fluid velocity at the wall is considered zero, mimicking the absence of relative motion between the fluid and the solid surface. This boundary condition is relevant for studying heat transfer, aerodynamic drag, and flow behavior around solid objects.
The Wall Condition is crucial in numerous applications, such as analysis of boundary layer formation, vehicle aerodynamics, and convective heat transfer. It allows engineers to study how fluid flow interacts with solid surfaces, enabling them to optimize designs and mitigate issues like drag, heat transfer inefficiencies, and structural loads.
The Symmetry Condition
The Symmetry Condition is applied when a physical domain possesses symmetrical properties. This boundary condition allows engineers to simulate only a portion of the entire geometry, resulting in reduced computational effort. Symmetry conditions are often utilized in models where the geometrical symmetry simplifies the analysis while still capturing the essential flow physics.
By applying the Symmetry Condition, CFD simulations can focus on the relevant regions, reducing computational resources and time. This is advantageous in cases such as analyzing aircraft components, electronic chip cooling, or fluid flow within rotating machinery. The Symmetry Condition enables engineers to gain insights into the behavior of the entire system while only simulating a portion of it.
The Periodic Condition
The Periodic Condition is employed when a flow phenomenon repeats itself in a defined periodic manner. It allows engineers to simulate only a portion of the whole domain, reducing computational costs while capturing the periodic behavior. This boundary condition is particularly useful when studying systems with repeating elements or understanding periodic oscillations.
By utilizing the Periodic Condition, engineers can analyze flow phenomena such as flow-induced vibrations, turbomachinery efficiency, or fluid-structure interactions. This condition allows for the study of dynamic responses of rotating components, optimizing designs, and predicting system performance under different operating conditions.
The Far Field Condition
The Far Field Condition is implemented to simulate flow characteristics in the far-reaching regions away from the boundaries of the computational domain. This boundary condition assumes that the flow properties at the boundaries do not significantly influence the flow in the far field, simplifying the simulation setup and reducing computational resources.
With the Far Field Condition, engineers can focus on analyzing flow behavior in regions where detailed knowledge of the boundary conditions is not required. This condition is often used in fluid-structure interaction simulations, large-scale environmental modeling, or analyzing aerodynamic flow around large structures such as buildings, bridges, or wind turbines.
Conclusions
In conclusion, defining appropriate boundary conditions is crucial when conducting real-world CFD simulations. The Mass Flow or Velocity Inlet Condition, Pressure Outlet Condition, Wall Condition, Symmetry Condition, Periodic Condition, and Far Field Condition are some of the most commonly used conditions in various industrial applications. By understanding and accurately specifying these conditions, engineers can accurately model and analyze fluid flow phenomena, optimize designs, and ensure efficient and reliable system performance.