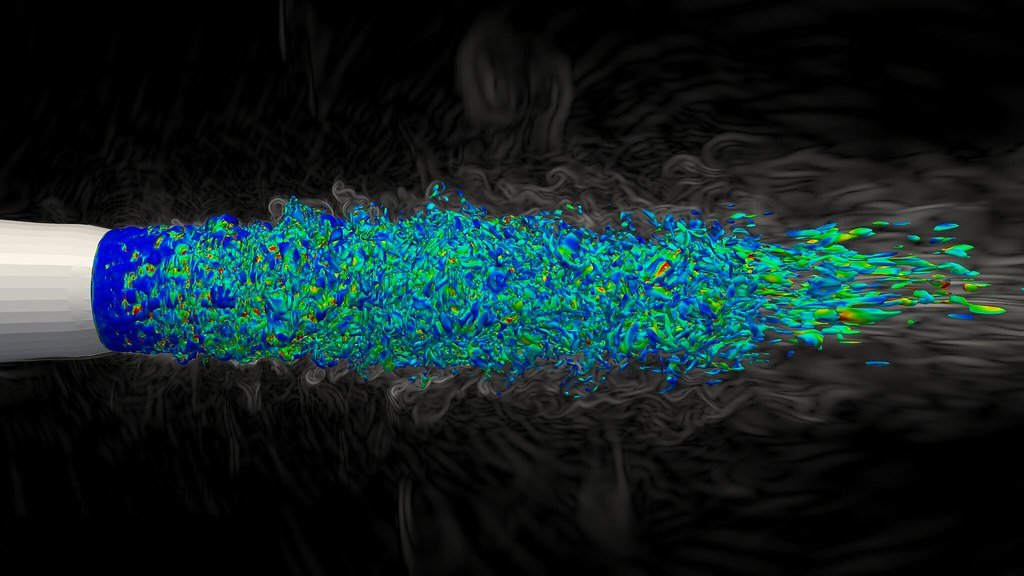
Introduction to Large Eddy Simulation (LES)
What is Large Eddy Simulation (LES)?
Large Eddy Simulation (LES) is a computational fluid dynamics (CFD) technique used to study and simulate turbulent flow in various engineering applications. Turbulence, characterized by chaotic and rapid fluid motion, often poses significant challenges when it comes to accurately predicting and modeling fluid behavior using traditional CFD methods. LES offers a promising alternative by providing a more detailed and realistic representation of turbulent flows.
The Challenge of Turbulence to CFD
One of the major hurdles faced by Computational Fluid Dynamics (CFD) is the accurate modeling of turbulence. Turbulent flows involve a wide range of length and time scales that span a vast range of orders of magnitude. The smallest eddies, known as the subgrid-scale (SGS) turbulence, interact with each other and with larger-scale motion, leading to complex flow phenomena.
Resolving the entire range of scales would require an excessively fine computational grid and enormous computational resources, making it impractical for most engineering applications. However, researchers have been tirelessly working on developing innovative solutions to tackle this challenge.
To address this issue, researchers developed a mathematical approach known as Reynolds-averaged Navier-Stokes (RANS) equations. RANS models average and filter out the small-scale turbulence, providing a simplified representation of the flow. This approach has been widely used in engineering applications, where the focus is on steady-state flows and where the effects of unsteady turbulence are not critical.
However, RANS has certain limitations, particularly when it comes to accurately capturing unsteady and coherent turbulence structures. This is where Large Eddy Simulation (LES) comes into play. LES is a computational technique that aims to resolve the large-scale turbulent structures while modeling the smaller-scale turbulence. It provides a more detailed and accurate representation of the flow compared to RANS.
In LES, the large-scale structures are directly resolved on the computational grid, while the smaller-scale turbulence is modeled using subgrid-scale models. This allows for a more realistic simulation of turbulent flows, capturing the dynamic behavior of the flow and its interaction with the surrounding environment.
LES has found applications in various fields, including aerospace, automotive, and environmental engineering. It has been used to study the aerodynamics of aircraft, the flow around vehicles, and the dispersion of pollutants in the atmosphere. By providing a more accurate representation of turbulent flows, LES enables engineers and scientists to make informed decisions and optimize designs.
Despite its advantages, LES still poses challenges in terms of computational cost and grid resolution. The computational resources required for LES simulations are significantly higher compared to RANS, as LES involves resolving a larger portion of the turbulent flow. Additionally, the grid resolution needs to be carefully chosen to ensure that the important turbulent structures are captured without excessive computational cost.
Researchers continue to explore new techniques and methodologies to improve the accuracy and efficiency of turbulence modeling in CFD. This includes the development of hybrid models that combine the strengths of RANS and LES, as well as the use of advanced numerical methods and high-performance computing.
In conclusion, the challenge of turbulence to CFD is a complex and ongoing research area. Accurately modeling turbulence is crucial for a wide range of engineering applications, and researchers are constantly pushing the boundaries to develop better and more efficient techniques. The advancements in turbulence modeling have significantly contributed to the understanding and prediction of complex flows, leading to improved designs and optimized performance in various industries.
Why an Alternative to Reynolds' Averaged Navier-Stokes was Needed
The limitations of Reynolds-Averaged Navier-Stokes (RANS) models in accurately simulating turbulent flows led researchers to seek an alternative approach that could provide a more realistic representation of turbulence. While RANS models have been widely used in engineering applications, they are based on the assumption of turbulence closure, which introduces certain simplifications and limitations.
RANS models average the turbulent flow variables over time, resulting in a time-averaged solution. This approach works well for steady or slowly varying flows, but it fails to capture the unsteady and highly turbulent nature of many practical flow situations. The inability of RANS models to accurately predict complex flow phenomena, such as vortex shedding, flow separation, and turbulent mixing, motivated the development of an alternative technique.
Large Eddy Simulation (LES) emerged as a promising technique that overcomes the limitations of RANS models. LES is a computational fluid dynamics (CFD) method that resolves the large-scale turbulent structures while modeling the small-scale turbulence. Unlike RANS models, LES does not rely on turbulence closure assumptions and provides a more direct and accurate representation of the turbulent flow field.
In LES, the large-scale turbulent structures are resolved on the computational grid, while the small-scale turbulence is modeled using a subgrid-scale (SGS) model. This allows for a more detailed and realistic simulation of the flow, capturing the energy-containing eddies that are responsible for most of the turbulence. By resolving the large-scale structures, LES provides a better prediction of flow features such as flow separation, vortices, and turbulent mixing.
The improved accuracy of LES comes at the cost of increased computational requirements compared to RANS models. Resolving the large-scale structures requires a finer grid resolution, which increases the computational cost of the simulation. However, advancements in computational resources and algorithms have made LES more feasible for practical engineering applications.
LES has been successfully applied to a wide range of flow problems, including turbulent boundary layers, jet flows, combustion processes, and aerodynamic flows over complex geometries. Its ability to capture the unsteady and highly turbulent nature of these flows has made LES a valuable tool for engineers and scientists in various fields.
In conclusion, the limitations of RANS models in accurately simulating turbulent flows led to the development of an alternative approach, known as Large Eddy Simulation (LES). LES provides a more realistic representation of turbulence by resolving the large-scale turbulent structures and modeling the small-scale turbulence. Although LES requires increased computational resources, its ability to capture complex flow phenomena has made it a valuable tool in engineering and scientific research.
LES - Low Pass Spatial Filtering Preserving Time Dependence
At the core of LES lies the concept of low pass spatial filtering, which allows for the selective representation of resolved scales while filtering out the subgrid-scale turbulence. This filtering helps in preserving the time-dependent behavior of the larger-scale turbulent structures, which are often of primary interest in many engineering applications.
The spatial filtering in LES is designed to resolve the larger energy-containing eddies, known as the resolved scales, while modeling the subgrid-scale turbulence using appropriate closure models. By explicitly simulating the larger turbulent structures and modeling the effect of the smaller scales, LES provides a more accurate prediction of flow characteristics.
LES in Practice
In practice, LES involves dividing the computational domain into a grid of cells, with each cell representing a discrete volume element. Various numerical methods, such as finite volume or finite difference schemes, are applied to solve the governing equations of fluid flow, incorporating the LES filtering operations.
LES has been successfully applied to a wide range of engineering problems, including aerodynamics, combustion, and environmental flows. It has proven to be particularly useful in scenarios where accurately capturing the details of turbulent flow structures is crucial for understanding the underlying physics and improving design performance.
Summary
In summary, Large Eddy Simulation (LES) is a powerful computational tool that allows for a more accurate modeling and simulation of turbulent flows. By resolving the larger turbulent structures and modeling the subgrid-scale turbulence, LES provides a detailed representation of flow behavior in various engineering applications. Its ability to capture the time-dependent behavior of turbulence makes it a valuable alternative to Reynolds-averaged Navier-Stokes (RANS) models, advancing our understanding of complex fluid dynamics and aiding in the design and optimization of engineering systems.