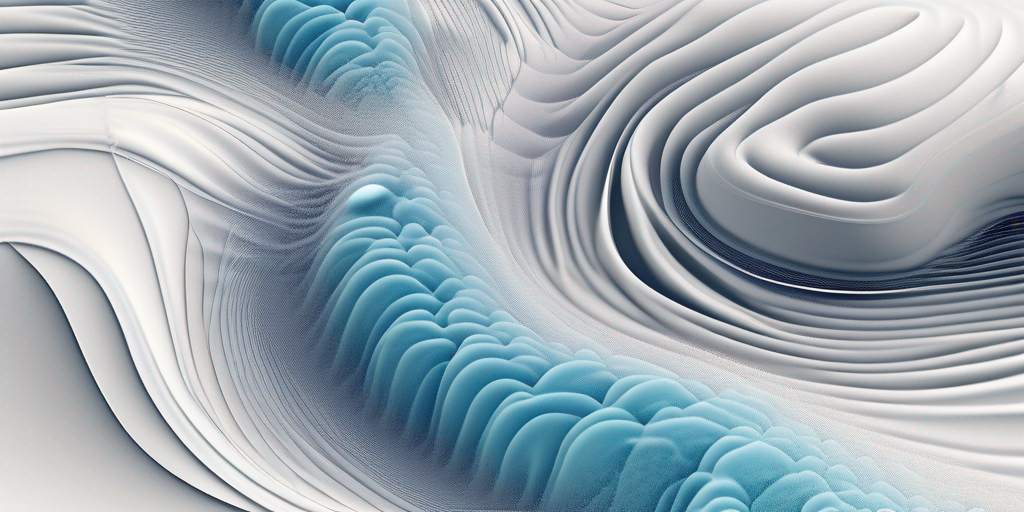
Modeling Phase Change Physics in CFD
An Introduction to Modeling Phase Change in CFD
Computational Fluid Dynamics (CFD) is a powerful tool used to simulate and analyze a wide range of fluid flow phenomena. It is widely employed in various industries, including aerospace, automotive, and energy, to study complex fluid behaviors and solve fluid flow problems. One particular aspect of fluid flow that is often encountered in these industries is phase change. In this article, we will explore the basics of phase change and discuss the models used to simulate phase change behavior in CFD.
What is a Phase?
Before we delve into phase change modeling, it is essential to understand what we mean by a phase. In thermodynamics, a phase refers to a distinct state of matter characterized by its physical properties, such as density, temperature, and pressure. The most commonly known phases are solid, liquid, and gas. These phases exist due to the different arrangements and energies of the particles that make up the substance.
Let's take a closer look at each of these phases:
What is a Solid? When a substance is in a solid phase, its particles are tightly packed together in a regular pattern. This arrangement gives solids a fixed shape and volume. Examples of solids include ice, wood, and metals.
What is a Liquid? In the liquid phase, the particles have more freedom to move compared to solids. They are still close together, but not as tightly packed. Liquids take the shape of their container and have a definite volume. Water, oil, and milk are common examples of liquids.
What is a Gas? Gases have particles that are far apart and move freely in all directions. They do not have a fixed shape or volume and can expand to fill any container. Examples of gases include air, helium, and carbon dioxide.
Now that we have a basic understanding of the different phases, let's explore how phase change occur. When considering phase changes, we are typically interested in the transition between these well-defined phases. This transition can occur when heat is added or removed from a substance, causing a change in temperature and, consequently, a change in phase.
Phase change phenomena are prevalent in various engineering applications. For example, in refrigeration systems, a liquid refrigerant undergoes phase change from a liquid to a gas in the evaporator, absorbing heat from the surroundings. On the other hand, in power plants, water is heated to produce steam, which drives turbines to generate electricity. These examples highlight the importance of understanding and accurately modeling phase change in engineering simulations.
Phase change Computational Fluid Dynamics (CFD) modeling involves complex mathematical equations that describe the behavior of the substance during the transition between phases. These equations take into account factors such as latent heat, which is the energy absorbed or released during phase change, and the rate at which the phase change occurs.
By accurately simulating phase change phenomena, engineers and scientists can gain insights into various processes, such as melting, freezing, boiling, and condensation. This knowledge can be applied to improve the design and efficiency of systems, ranging from refrigeration and air conditioning to chemical reactors and combustion engines.
As technology advances, the ability to model phase change becomes increasingly important in fields such as aerospace, automotive, and energy. Researchers are continuously developing new algorithms and computational techniques to enhance the accuracy and efficiency of phase change simulations.
What are the Types of Phase Changes?
In the field of thermodynamics, there are several types of phase changes that we commonly encounter. These include:
Fusion/Melting:
The transition from a solid to a liquid phase. This occurs when the substance absorbs enough heat to overcome the forces binding the particles in the solid phase. Fusion, also known as melting, is a fascinating process that occurs when a substance transitions from a solid to a liquid phase. Imagine a block of ice sitting on a table. As heat is applied to the ice, the temperature of the ice gradually increases. At a certain point, known as the melting point, the ice begins to transform into water. This is because the ice particles absorb enough heat energy to overcome the forces that hold them in a rigid, solid structure. The particles gain enough kinetic energy to break free from their fixed positions and start moving more freely, resulting in the formation of a liquid.
Vaporization/Boiling:
The transition from a liquid to a gas phase. This occurs when the substance absorbs enough heat to break the intermolecular forces between the liquid particles. Vaporization, commonly known as boiling, is a process in which a liquid substance transforms into a gas phase. Think of a pot of water being heated on a stove. As the water is heated, its temperature rises. Eventually, the water reaches its boiling point, and bubbles start to form and rise to the surface. This is because the liquid particles gain enough energy to break the intermolecular forces that hold them together. The transition from a liquid to a gas phase is accompanied by an increase in volume and the formation of vapor.
Condensation:
The transition from a gas to a liquid phase. This occurs when the substance releases enough heat to form intermolecular bonds between the gas particles. Condensation is the reverse process of vaporization. It happens when a gas cools down and transforms into a liquid phase. Picture a cold glass of water on a hot summer day. As the warm air around the glass comes into contact with its cold surface, the water vapor in the air loses heat energy and begins to condense. This is because the gas particles lose enough energy to form intermolecular bonds with each other, resulting in the formation of tiny droplets of liquid on the surface of the glass.
Sublimation:
The transition from a solid to a gas phase without passing through the liquid phase. This occurs when the substance absorbs enough heat to overcome the forces binding the particles in the solid phase directly into the gas phase. Sublimation is a unique phase change process that bypasses the liquid phase altogether. It occurs when a solid substance is heated and transforms directly into a gas phase. A common example of sublimation is dry ice, which is solid carbon dioxide. When exposed to room temperature, dry ice undergoes sublimation, transforming from a solid directly into a gas, without melting into a liquid first. This happens because the solid particles gain enough energy to break free from their fixed positions and transition into the gas phase.
Deposition:
The transition from a gas to a solid phase without passing through the liquid phase. This occurs when the substance releases enough heat to form intermolecular bonds between the gas particles directly into the solid phase. Deposition is the reverse process of sublimation. It takes place when a gas directly transforms into a solid phase without going through the liquid phase. An example of deposition is the formation of frost on a cold surface. When moist air comes into contact with a cold window pane, the water vapor in the air loses heat energy and undergoes deposition, transforming directly into ice crystals on the surface. The gas particles lose enough energy to form intermolecular bonds with each other, resulting in the solidification of the gas into a solid phase.
Understanding these different types of phase changes is crucial to accurately model phase change behavior in CFD simulations. By considering the specific characteristics and energy requirements of each phase change, scientists and engineers can develop more precise models to study and predict the behavior of substances under different conditions.
Models of Phase Change Behavior in CFD
Modeling phase change behavior in CFD involves developing mathematical models that simulate the physical processes occurring during phase change. These models take into account factors such as heat transfer, mass transfer, and the thermodynamic properties of the substances involved.One commonly used model is the enthalpy-porosity method. This approach represents the phase change interface as a transition zone with varying porosity, which allows for the exchange of heat and mass between the phases. The enthalpy, a measure of the internal energy of a system, is utilized to account for the energy transfer during phase change.
Another widely employed model is the volume of fluid (VOF) method. This method tracks the movement of fluid interfaces within a computational domain. By modeling the location and shape of the interface, the VOF method enables accurate representation of phase change phenomena, including the formation and disappearance of fluid interfaces.
Additionally, the phase field method is gaining popularity in phase change simulations. This method describes the phase change process using a scalar field variable, known as the phase field. The phase field variable represents the degree of phase transformation and evolves according to a set of governing equations.
Each of these modeling approaches has its own advantages and limitations, and the choice of model depends on the specific phase change scenario and computational requirements.
Conclusion
In conclusion, phase change modeling plays a pivotal role in simulating and understanding the behavior of fluids in various industrial applications. By accurately capturing the physical processes involved in phase change, CFD simulations can provide valuable insights and aid in the design and optimization of systems involving phase change phenomena.
Whether it's analyzing the melting of a solid fuel in rocket propulsion or simulating the boiling of a coolant in a nuclear reactor, the ability to model phase change behavior in CFD simulations is key to advancing engineering solutions. As research continues to advance, we can expect further developments in the field of phase change modeling, enabling more accurate and efficient simulations.