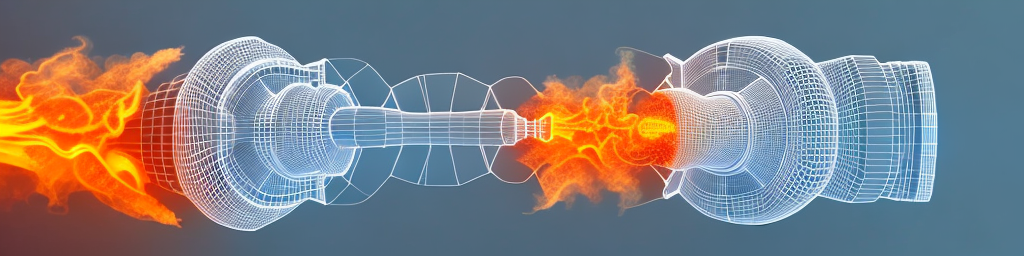
Introduction to CFD Combustion Models
Exploring Combustion Models in CFD
Computational Fluid Dynamics (CFD) is a rapidly advancing field responsible for simulating fluid flow and heat transfer across complex systems. In recent years, the field has made significant progress in predicting combustion phenomena. As a result, CFD models have become an essential tool for the design and optimization of combustion systems.
The Basics of Combustion
Combustion is a fascinating phenomenon that has been studied for centuries. It is a chemical reaction that occurs between a fuel and an oxidizer, resulting in the release of energy in the form of heat and light. The combustion process can be observed in everyday life, from the flame of a candle to the roar of a rocket engine.
Before diving into the various CFD models for combustion, it's essential to understand the basics of combustion. The process of combustion can be divided into three stages: ignition, flame propagation, and flame extinction.
Ignition
Ignition is the initial stage of combustion where a flame is ignited from a spark, hot surface, or by preheating the gases. This stage is critical because it determines whether the combustion process will continue or not. For example, in an internal combustion engine, the spark plug ignites the fuel-air mixture, which then burns and produces the energy needed to power the engine.
However, ignition can also occur spontaneously in certain conditions, such as in diesel engines, where the high pressure and temperature of the compressed air is enough to ignite the fuel without a spark plug.
Flame Propagation
Once ignition occurs, the flame propagation stage begins. This is where the flame spreads throughout the fuel mixture. The rate of flame propagation depends on various factors, such as the fuel type, fuel-air ratio, and temperature. In some cases, the flame can propagate at supersonic speeds, creating a shock wave known as a detonation.
Flame propagation can also be affected by turbulence in the mixture. Turbulence can increase the surface area of the fuel and air, allowing for a faster and more efficient combustion process. This is why many combustion systems, such as gas turbines, use swirl or other flow control devices to enhance turbulence.
Flame Extinction
The final stage of combustion is flame extinction. This occurs when the flame is extinguished due to lack of fuel or air, or other factors such as quenching by a solid surface or by the injection of an inert gas. In some cases, flame extinction can be desirable, such as in fire suppression systems or when extinguishing a controlled burn.
However, in most cases, flame extinction is unwanted and can lead to incomplete combustion or even explosions. Therefore, it's crucial to understand the factors that can cause flame extinction and design combustion systems that prevent it from occurring.
Overall, combustion is a complex process that involves many factors and variables. Understanding the basics of combustion is essential for designing efficient and safe combustion systems, as well as for developing advanced CFD models that accurately simulate combustion processes.
Premixed Vs. Diffusion Flames
In the field of combustion, the study of premixed and diffusion flames is of utmost importance. Premixed flames are those in which the fuel and oxidizer are mixed together before combustion occurs. This type of flame is commonly used in gas stoves, as the fuel and air are mixed together in the burners before ignition. On the other hand, diffusion flames occur when the fuel and oxidizer mix as they react. A candle flame is a great example of a diffusion flame, as the wax vaporizes and mixes with the surrounding air, creating a flame.
When it comes to CFD modeling, the differences between premixed and diffusion flames are significant. In premixed flames, the flame front speed is relatively constant, meaning that the flame propagates at a constant rate. However, in diffusion flames, the flame front speed varies according to the local fuel and oxidizer concentrations. This is because the fuel and oxidizer are not uniformly mixed, which leads to variations in the reaction rate and, therefore, the flame speed.
It is important to note that the combustion process is complex and can be affected by a variety of factors, including temperature, pressure, and chemical composition. In premixed flames, the fuel and oxidizer are already mixed, which means that the combustion process is more controlled and predictable. In diffusion flames, however, the combustion process is more complex and can be affected by factors such as turbulence and mixing.
In conclusion, while both premixed and diffusion flames are important in the field of combustion, they differ significantly in terms of their combustion processes and CFD modeling. Understanding these differences is crucial for researchers and engineers working in the field of combustion, as it can help them design more efficient and effective combustion systems.
Flamelet Models Defined
Flamelet models are a type of combustion model that describes the behavior of flames by assuming that the flame is a thin layer near the fuel-oxidizer interface. This approach simplifies the description of the reaction kinetics by assuming that the flame is in local thermodynamic equilibrium. Flamelet models are widely used in combustion simulations due to their computational efficiency and accuracy in predicting turbulent flames.
However, flamelet models come in different forms, each with its own set of assumptions and benefits. In this article, we will discuss three popular flamelet models: the Flamelet Generated Manifold (FGM) model, the Steady Laminar Flamelet (SLF) model, and the Chemical Equilibrium (CE) model.
Details on Flamelet Generated Manifold Model
The Flamelet Generated Manifold (FGM) model is a widely used flamelet approach that represents the flame as a function of the fuel-oxidizer-ratio and temperature. The solution is then interpolated during simulation, making it computationally efficient. The FGM model is particularly useful for simulating turbulent flames, and it can be extended to account for different combustion modes and dynamic effects.
One of the benefits of the FGM model is that it provides a good balance between accuracy and computational cost. It is also versatile and can be used for a variety of combustion systems. However, the FGM model assumes that the flame is in local thermodynamic equilibrium, which may not always be the case in real-world scenarios.
Details on the Steady Laminar Flamelet Model
The Steady Laminar Flamelet (SLF) model is a more complex flamelet model that explicitly solves the flow and flame equations for laminar flames. This approach provides significant details of the flame structure, enabling better optimization of combustion systems. However, the SLF model is computationally intensive and is typically used for simulating simple flames.
The SLF model is particularly useful for studying the effects of flame stretch and strain rate on combustion. It can also be used to investigate the impact of different parameters, such as fuel composition and inlet conditions, on the flame structure. However, the SLF model may not be suitable for simulating complex combustion systems due to its high computational cost.
Details on the Chemical Equilibrium Model
The Chemical Equilibrium (CE) model is an analytical approach used to describe the chemical reactions during combustion. It assumes that the reaction is at thermodynamic equilibrium, allowing for a simplified description of the reaction kinetics. The CE model is particularly useful for predicting equilibrium composition and temperature in combustion chambers.
The CE model is computationally efficient and can provide valuable insights into the combustion process. However, it may not be suitable for simulating non-equilibrium combustion systems, where the reaction kinetics may deviate significantly from thermodynamic equilibrium.
Overall, flamelet models are a useful tool for simulating combustion systems. Each model has its own set of assumptions and benefits, and the choice of model depends on the specific application and computational resources available.
Flame Position Models Defined
Flame Position Models (FPMs) model combustion by simulating the position of the flame front. FPMs are often used in conjunction with other CFD models for simulating more complex combustion processes.
One of the major advantages of FPMs is that they can be used to predict the flame position and shape, which is critical for understanding the combustion process. FPMs can also be used to study the effects of different fuel types, burner designs, and operating conditions on flame position and stability.
Another important aspect of FPMs is that they can be used to optimize combustion processes. By simulating different operating conditions and fuel types, engineers can identify the most efficient and effective way to burn fuel, which can lead to significant cost savings and reduced emissions.
Flamelet Tables Defined
An essential aspect of flamelet models is the Flamelet Table (FT). It is a precomputed database of the flame structure and thermodynamic properties. The FT is interpolated during simulation to provide a fast and accurate description of the flame position and properties.
Flamelet tables are widely used in combustion modeling because they provide a computationally efficient way to simulate complex combustion processes. By precomputing the flame structure and properties, the simulation time can be greatly reduced, making it possible to study complex combustion processes in real-time.
Flamelet tables are also useful for studying the effects of different fuel types and operating conditions on combustion. By changing the input parameters in the flamelet table, engineers can simulate different combustion scenarios and identify the most efficient and effective way to burn fuel.
Overall, flamelet models and flamelet tables are powerful tools for studying combustion processes and optimizing combustion systems. They provide a fast and accurate way to simulate complex combustion processes, which can lead to significant cost savings and reduced emissions.
Conclusion
In conclusion, computational fluid dynamics has revolutionized the study and optimization of combustion systems. The various flamelet and flame position models utilized in CFD provide a comprehensive description of combustion phenomena. From the Chemical Equilibrium model to the Flamelet Generated Manifold model, each approach provides unique insights into the complex world of combustion.