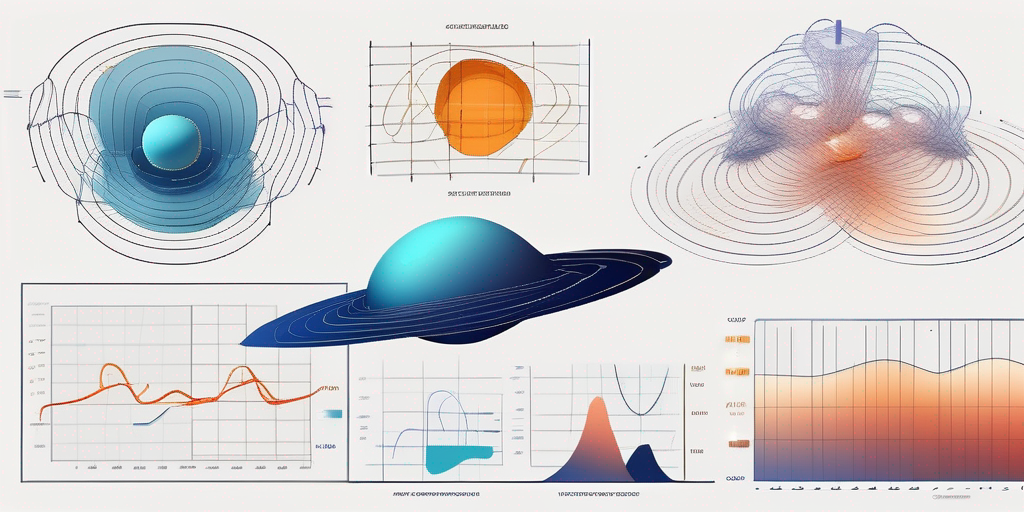
An Introduction to Selecting CFD Physics Models
How to Select the Right Physics Models to Use in CFD
Computational Fluid Dynamics (CFD) is a powerful tool used in various industries to simulate fluid flow and understand complex phenomena. To accurately represent these flows, choosing the appropriate physics models is crucial. This article aims to guide you through the process of selecting the right physics models for your CFD simulations, taking into account various considerations. By understanding the basics of physics models, evaluating factors, and overcoming potential challenges, you can enhance the accuracy and reliability of your CFD results.## Understanding the Basics of Physics Models in CFD
Before diving into the selection process, it is essential to grasp the significance of physics models in Computational Fluid Dynamics (CFD). Physics models define the mathematical equations and assumptions employed to represent fluid flow behavior. They encompass a wide range of physical phenomena, such as turbulence, combustion, heat transfer, and multiphase flows, depending on the complexity of the problem at hand.
In CFD, physics models enable the simulation of real-world scenarios by simplifying and approximating the underlying physics. Understanding the role they play in shaping the flow behavior is vital for accurate and efficient simulations.
When it comes to CFD simulations, physics models are like the building blocks that allow engineers to recreate and analyze fluid flow phenomena. These models are based on fundamental principles of fluid mechanics and transport phenomena, which are then translated into mathematical equations. By solving these equations numerically, CFD practitioners can obtain predictions of flow patterns and other important flow characteristics.
Defining Physics Models and CFD
In the context of CFD, physics models mathematically describe the fluid flow behavior and its interaction with various physical aspects, such as solids, heat, and turbulence. These models rely on fundamental principles of fluid mechanics and transport phenomena to simulate and predict flow patterns.
CFD, on the other hand, is the branch of computational science that deals with solving the governing equations of fluid flow numerically. It involves discretizing the fluid domain into a computational grid, applying appropriate boundary conditions, and solving the equations iteratively to obtain flow field variables.
By combining physics models with CFD techniques, engineers can gain valuable insights into the behavior of fluid flows in a wide range of applications. Whether it's analyzing the aerodynamics of an aircraft, optimizing the performance of a heat exchanger, or studying the flow behavior in a chemical reactor, physics models play a crucial role in accurately representing the complex phenomena involved.
The Role of Physics Models in CFD
Physics models serve as the backbone of any CFD simulation. They enable engineers to predict and analyze complex flow phenomena that are otherwise difficult or impossible to test experimentally. By selecting suitable physics models, CFD practitioners can accurately capture the essential characteristics of the flow, leading to valuable insights and informed decision-making.
However, it is crucial to recognize that physics models are simplifications of reality and come with inherent limitations. Selecting an appropriate model involves striking a balance between computational efficiency and accuracy, depending on the specific requirements of the problem.
For example, in turbulent flows, there are various turbulence models available, each with its own level of complexity and accuracy. Choosing the right turbulence model requires considering factors such as computational resources, time constraints, and the desired level of accuracy. It is a trade-off between accuracy and computational cost.
Furthermore, the selection of physics models also depends on the specific flow regime being studied. For instance, if the flow involves heat transfer, appropriate models for convection, conduction, and radiation need to be selected to accurately capture the energy transfer mechanisms.
In conclusion, physics models are an integral part of CFD simulations, allowing engineers to simulate and analyze complex flow phenomena. By understanding the role of physics models in CFD, practitioners can make informed decisions when selecting appropriate models, considering the trade-offs between accuracy and computational efficiency.
Key Factors to Consider When Selecting Physics Models
The Nature of Your CFD Problem
The first step in selecting physics models is understanding the nature of your CFD problem. Consider the type of flow (e.g., laminar or turbulent), whether the flow is compressible or incompressible, the presence of heat transfer or multiphase phenomena, and any other relevant aspects.
Each physics model excels in particular flow scenarios and may exhibit limitations in others. By aligning the physics model with your problem's characteristics, you can ensure accurate representation and reliable results.
The Complexity of the Model
Physics models can vary in complexity, from simple algebraic relations to computationally intensive equations. The choice of model complexity should be based on the intended use of the simulations and the computational resources available.
Complex models may provide high-fidelity results but require significant computational power and time. Simpler models, on the other hand, offer computational efficiency but may sacrifice accuracy. Balancing complexity with available resources is crucial to strike an optimal trade-off.
The Accuracy Required
Different applications have varying requirements for accuracy. Some simulations demand precise predictions, such as aerospace or medical applications, while others can tolerate reasonable approximations. Understanding the level of accuracy required is crucial in selecting the appropriate physics model.
High-accuracy models often entail more computational effort. However, it is essential to evaluate whether the increased accuracy justifies the additional time and resources or if a less accurate model can deliver sufficient insights.
Common Physics Models Used in CFD
Laminar Flow Models
Laminar flow models assume that the fluid flows smoothly and in an organized manner, with minimal turbulence. These models are suitable for low-speed or highly viscous flows, where turbulence effects are negligible. They are commonly used in applications such as microfluidics, lubrication analysis, or flows with a high degree of rotational symmetry.
Using laminar flow models can significantly reduce computational costs compared to more complex turbulent models, making them a practical choice in specific scenarios.
Turbulent Flow Models
Turbulent flow models address flows with high levels of turbulence, where the fluid exhibits chaotic and irregular motion that significantly impacts macroscopic properties. Turbulence occurs in many practical cases, such as pipe flows, airfoils, or environmental flows.
Turbulent models range from simple empirical relations, such as the widely-used k-epsilon model, to more sophisticated large eddy simulation (LES) or detached eddy simulation (DES) models. The choice of turbulent model depends on the flow characteristics and desired accuracy.
The Process of Selecting the Right Physics Model
Identifying Your Needs
The selection process starts with a clear identification of your requirements and constraints. Define the objectives of your simulation, considering factors such as accuracy, available computational resources, and computational time constraints.
Identifying these needs upfront will guide your selection process, allowing you to narrow down the choices and focus on the most suitable physics models for your specific application.
Evaluating Different Models
Once your needs are defined, consider evaluating multiple physics models. This assessment typically involves comparing the model's predictive performance against experimental or benchmark data or utilizing existing knowledge and literature.
Validate the models against known cases or test scenarios relevant to your application. Look for models that exhibit good agreement with experimental or established results, demonstrating their reliability and accuracy.
Implementing the Selected Model
After selecting the appropriate physics model, incorporate it into your CFD solver or commercial software. Familiarize yourself with the model's setup requirements, including required input parameters, boundary conditions, and solver settings.
Ensure compatibility between the chosen model and the software platform you are using, verifying that the solver supports the selected model and its associated equations.
Potential Challenges in Selecting Physics Models
Overcoming Computational Limitations
Certain physics models may pose significant computational challenges due to their complexity or resource requirements. High-fidelity models, such as large eddy simulation or direct numerical simulation, demand substantial computational power to capture intricate flow features accurately.
If computational limitations exist, consider alternative models or simplifications that strike a balance between computational efficiency and desired accuracy. Cautiously assess the trade-offs between accuracy and computational resources to optimize your simulations.
Dealing with Model Uncertainty
Physics models inherently involve simplifications and assumptions that introduce uncertainty into the simulations. This uncertainty arises from uncertainties in model coefficients, boundary conditions, or simplifications of complex physical phenomena.
It is essential to understand and quantify the sources and impact of model uncertainty on your results. By conducting sensitivity analyses and uncertainty quantification, you can assess the reliability of the selected model and determine its limitations.
Conclusions
In conclusion, selecting the right physics models for CFD simulations requires careful consideration of factors such as the problem's nature, complexity, and required accuracy. By understanding the basics of physics models, evaluating and comparing various options, and addressing potential challenges, you can make informed decisions that enhance the validity and usefulness of your CFD simulations.