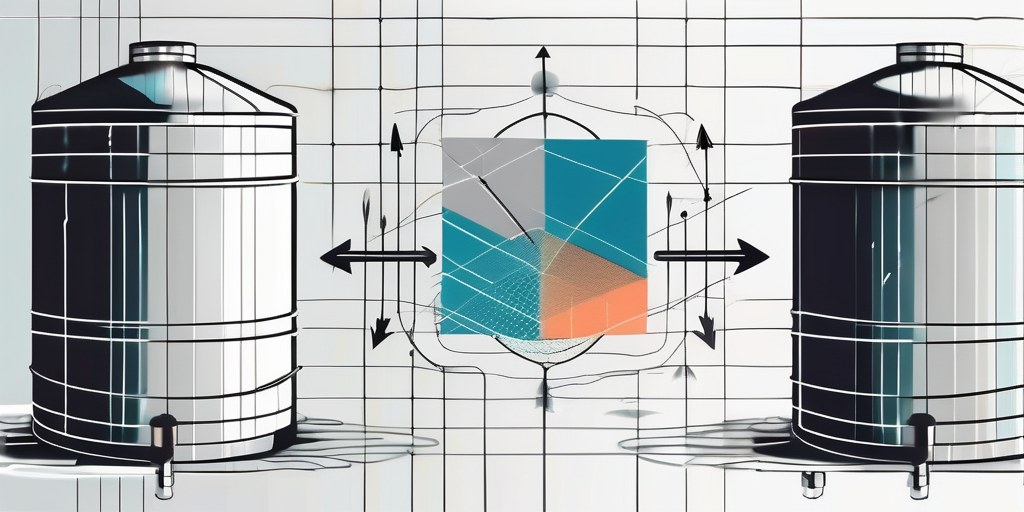
Improving Municipal Water Quality with Computational Fluid Dynamics
CFD for Water Tank Circulation
In the world of water tank management, one of the key challenges is maintaining optimal water quality. Thermal stratification, the layering of water temperatures in a tank, can significantly impact the growth of microbes and the formation of disinfection byproducts (DBPs). To prevent such issues, active mixing is crucial. This is where computational fluid dynamics (CFD) comes into play, offering a powerful tool for optimizing water tank circulation.
Thermal Stratification Accelerates Microbial and Disinfection Byproduct Growth
Thermal stratification, a natural occurrence in water tanks, is a fascinating phenomenon that occurs when the water separates into distinct layers based on temperature. The warmest water tends to accumulate near the top of the tank, while the coldest water settles at the bottom. This separation can have significant implications for water quality and the formation of disinfection byproducts (DBPs).
When thermal stratification occurs, it creates an environment that is conducive to the growth of bacteria, algae, and other microorganisms. The upper layer, where the warmest water resides, provides the ideal conditions for these organisms to thrive. The increased temperature and exposure to sunlight in this region create a perfect breeding ground, leading to a decline in water quality.
Not only does thermal stratification promote the growth of microorganisms, but it also accelerates the formation of disinfection byproducts. DBPs, such as trihalomethanes and haloacetic acids, are generated when disinfectants, like chlorine, react with organic matter present in the water. The warmer temperatures in the upper layer of the tank can significantly increase the rate at which these compounds form, posing potential health risks to consumers.
It is important to note that the presence of thermal stratification is not limited to water tanks alone. This phenomenon can also occur in natural bodies of water, such as lakes and reservoirs. In these settings, thermal stratification can have profound effects on the ecosystem, disrupting the balance of aquatic life and altering water chemistry.
To mitigate the negative impacts of thermal stratification, various strategies can be employed. One approach is to implement tank mixing systems that circulate the water, preventing the formation of distinct layers. By continuously mixing the water, the temperature gradient is minimized, reducing the likelihood of microbial growth and DBP formation.
Another method to combat thermal stratification is through the use of thermal destratification devices. These devices help to equalize the temperature throughout the tank by redistributing the warm water from the top to the bottom and vice versa. By maintaining a more uniform temperature profile, the growth of microorganisms can be controlled, and the formation of DBPs can be minimized.
Understanding the complex relationship between thermal stratification, microbial growth, and DBP formation is crucial for ensuring the safety and quality of our water supply. Ongoing research and advancements in water treatment technologies are essential in developing effective strategies to mitigate the negative impacts of thermal stratification and protect public health.
Active Mixing Needed to Prevent Thermal Stratification
To mitigate thermal stratification, active mixing is essential. This process involves the controlled movement of water within the tank to prevent the buildup of stagnant zones and promote temperature uniformity throughout. By avoiding or minimizing thermal gradients, the growth of microbes and the formation of disinfection byproducts (DBPs) can be significantly reduced.
Active mixing systems utilize various mechanisms to achieve efficient water circulation. One common approach is the use of strategically placed impellers or mixers that create turbulence and induce fluid motion. These devices can be designed to operate at different speeds and directions, ensuring thorough mixing of the water in the tank.
Traditionally, the design and optimization of water tank circulation systems were based on empirical formulas and simplified models. However, these approaches often fell short in accurately predicting the complex fluid behavior occurring within the tank. Factors such as tank geometry, inlet and outlet locations, and the presence of obstacles can significantly influence the flow patterns and temperature distribution.
Recognizing the limitations of traditional methods, computational fluid dynamics (CFD) has emerged as a powerful tool for analyzing and optimizing active mixing systems. CFD simulations enable engineers to simulate the fluid flow and heat transfer processes within the tank, taking into account the intricate details of the system. By solving the governing equations numerically, CFD provides a detailed understanding of the flow patterns, temperature distribution, and residence time of the water.
With the aid of CFD, engineers can evaluate different active mixing strategies and optimize the design parameters to achieve the desired level of thermal uniformity. By simulating various scenarios and analyzing the results, they can identify potential issues such as dead zones or areas with excessive turbulence. This information allows for the refinement of the mixing system design, ensuring efficient and effective thermal stratification prevention.
In addition to preventing thermal stratification, active mixing systems offer other benefits. By promoting uniform temperature distribution, they enhance the performance of temperature-sensitive processes that rely on consistent water temperatures. This is particularly important in industries such as pharmaceuticals, food and beverage, and chemical processing.
Furthermore, active mixing can help prevent the accumulation of sediments and suspended solids in the tank. The turbulent flow created by the mixing devices helps to suspend and disperse particles, preventing their settling and reducing the need for frequent tank cleaning and maintenance.
Overall, active mixing plays a crucial role in maintaining optimal water quality and preventing the negative consequences of thermal stratification. Through the use of advanced techniques like CFD, engineers can design and optimize efficient mixing systems that ensure uniform temperature distribution, minimize the formation of DBPs, and enhance the overall performance of water storage and processing systems.
Optimizing Circulation with CFD
Computational fluid dynamics provides a detailed numerical analysis of fluid flow, heat transfer, and related phenomena within a water tank. This powerful tool enables engineers to simulate the behavior of water under various operating conditions, making it invaluable for optimizing circulation systems.
By utilizing CFD simulations, engineers can investigate different mixing methods and their effectiveness in preventing thermal stratification. These simulations can accurately predict the flow patterns, temperature distribution, and velocity profiles within the water tank. Armed with this information, engineers can then make informed design decisions to enhance water tank circulation and minimize the risks associated with thermal stratification.
One important aspect that CFD simulations can help with is the evaluation of the impact of different tank geometries on circulation. Engineers can explore the effects of tank shape, size, and internal structures on the flow behavior. For example, they can analyze the impact of tank baffles or flow control elements on the flow patterns and mixing efficiency. This allows engineers to optimize the tank design to achieve the desired circulation performance.
Furthermore, CFD simulations allow for the evaluation of potential modifications or additions to the system, ensuring that any adjustments made will result in improved performance. This can include the positioning and selection of mixing devices, the optimization of their operating parameters, or the introduction of baffles and flow control elements.
Another advantage of using CFD for optimizing water tank circulation is the ability to consider real-world operating conditions. Engineers can simulate the effects of different flow rates, inlet and outlet configurations, and even external factors such as ambient temperature and humidity. This comprehensive analysis helps to ensure that the circulation system is robust and capable of performing optimally under a wide range of conditions.
By harnessing the capabilities of CFD, engineers can gain valuable insights into the behavior of water tank circulation systems. With a deeper understanding of the complex fluid dynamics at play, they can develop solutions that optimize water quality, minimize the formation of disinfection byproducts, and ensure the safety and satisfaction of consumers.
Conclusions
CFD has emerged as a powerful tool for optimizing water tank circulation. By mitigating thermal stratification and promoting temperature uniformity, active mixing can significantly reduce microbial growth and the formation of disinfection byproducts. With the ability to simulate and analyze fluid behavior within a tank, CFD empowers engineers to make informed design decisions that enhance water quality and guarantee consumer safety. As our understanding of fluid dynamics continues to advance, CFD will undoubtedly play an increasingly pivotal role in revolutionizing water tank management.