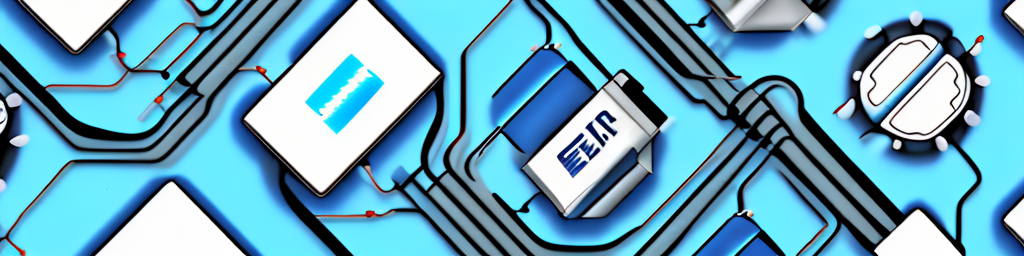
Optimizing Energy Storage: The Role of Computational Electro-Chemistry in Fuel Cell and Battery Designs
Effective Fuel Cell and Battery Designs Require Computational Electro-Chemistry Analyses
Fuel cells and batteries play a crucial role in our daily lives, powering everything from electric vehicles to electronic devices. To ensure optimal performance and longevity, it is imperative to understand the electro-chemical processes that occur within these energy storage devices. This is where computational electro-chemistry comes into play, providing a deeper understanding of the underlying science and enabling the development of more effective fuel cell and battery designs.
Understanding the Importance of Computational Electro-Chemistry in Energy Storage
At its core, electro-chemistry is the study of the relationship between electrical energy and chemical reactions. In the context of fuel cells and batteries, this involves the transfer of electrons through various materials and electrolytes. Computational electro-chemistry applies advanced mathematical models and simulations to provide insight into these processes and their impact on performance.
One of the most important applications of electro-chemistry is in energy storage. With the increasing demand for renewable energy sources, efficient and effective energy storage is becoming more and more important. Fuel cells and batteries are two of the most widely used methods of energy storage, and both rely heavily on electro-chemistry to function.
The Role of Electro-Chemistry in Fuel Cells and Batteries
Electro-chemistry is central to the functioning of fuel cells and batteries, which rely on the movement of ions and electrons to generate and store energy. In fuel cells, chemical reactions cause a flow of electrons between electrodes, generating electrical energy. In batteries, an electro-chemical reaction within the cell produces a flow of electrons between electrodes, storing electrical energy.
One of the key factors in the performance of fuel cells and batteries is the efficiency of the electro-chemical reactions that take place. Computational electro-chemistry allows researchers to study these reactions in detail, and to identify ways to improve their efficiency. This can lead to the development of more efficient and effective energy storage devices, which are crucial for the transition to a more sustainable energy system.
Advancements in Computational Electro-Chemistry Techniques
Recent advancements in computational electro-chemistry have led to more accurate and comprehensive models of energy storage devices. These models can simulate everything from the distribution of ions and electrons, to the behavior of specific materials and molecules within the device. This allows for more precise analysis of the factors that impact device performance, and enables the development of new, innovative designs.
One area where computational electro-chemistry is particularly useful is in the development of new materials for energy storage devices. By simulating the behavior of different materials under different conditions, researchers can identify materials that are likely to be effective in energy storage applications. This can save time and resources in the development process, and can lead to the discovery of new materials that may not have been identified through traditional experimental methods.
Overall, computational electro-chemistry is a crucial tool in the development of more efficient and effective energy storage devices. By providing detailed insights into the electro-chemical processes that underpin fuel cells and batteries, it enables researchers to identify ways to improve their performance and to develop new, innovative designs. As the demand for renewable energy sources continues to grow, the importance of computational electro-chemistry in energy storage will only increase.
Key Components of Fuel Cell and Battery Design
Optimizing fuel cell and battery performance requires detailed analysis of each component that makes up these devices. Here are some of the key components that must be examined in any electro-chemical analysis:
Electrodes and Their Material Properties
Electrodes are critical components in fuel cells and batteries that provide a surface for the transfer of electrons. Computational electro-chemistry can examine the properties of the electrode material, its physical characteristics, and its reaction with the surrounding electrolyte. This information is critical for understanding the efficiency and durability of the electrode and how it contributes to the overall performance of the device.
Electrolytes and Ion Transport
The electrolyte is the conductive medium that allows ions to move within the fuel cell or battery. Computational electro-chemistry can simulate and analyze the behavior of ions and electrons within the electrolyte to determine factors that may impact performance, such as ionic strength and conductivity.
Membranes and Separators
Membranes and separators are essential for isolating the anode from the cathode and allowing ions to move through the device. Computational electro-chemistry can study the mechanics of these components, including their porosity, ion selectivity, and reaction with other materials to identify areas for improvement.
Computational Methods for Electro-Chemical Analysis
Advanced computational methods are used to model and simulate electro-chemical reactions, enabling scientists and engineers to analyze and optimize fuel cell and battery designs. Here are some of the most commonly used methods:
Density Functional Theory (DFT)
DFT is a quantum mechanical modeling approach that can simulate the electronic structure and behavior of molecules and materials. This enables researchers to study the chemical reactions that occur within fuel cells and batteries and understand the factors that affect performance.
Molecular Dynamics Simulations
Molecular dynamics simulations track the movements of individual atoms within a system, providing insight into how molecules and materials interact with each other. For fuel cells and batteries, this can help scientists understand how ions move through the electrolyte and how electrodes and other components interact.
Finite Element Analysis (FEA)
FEA is a numerical method for solving equations that model the behavior of complex systems. It is often used to model and optimize the design of components in fuel cells and batteries and identify areas for improvement.
Case Studies: Successful Applications of Computational Electro-Chemistry
Computational electro-chemistry has already led to significant improvements in fuel cell and battery technology. Here are a few examples:
Improving Energy Density in Lithium-Ion Batteries
Researchers have used computational modeling to optimize the microstructure of lithium-ion battery electrodes, resulting in an increase in energy density and improved performance.
Enhancing the Efficiency of Proton Exchange Membrane Fuel Cells
Proton exchange membrane fuel cells are highly efficient energy storage devices, but they are also sensitive to changes in environmental conditions. Computational modeling has been used to optimize the design and materials of the proton exchange membrane, improving the performance and stability of these devices.
Developing Novel Electrode Materials for Supercapacitors
Supercapacitors are a promising energy storage technology that can store and release energy quickly. Computational modeling has been used to develop novel electrode materials for supercapacitors, improving their efficiency, power density, and cycle life.
Conclusion
Computational electro-chemistry is an essential tool for designing and optimizing fuel cells and batteries. Through advanced modeling and simulation techniques, researchers can gain a deeper understanding of the electro-chemical processes occurring within these devices and identify areas for improvement. This has led to significant advancements in energy storage technology and will continue to be an important area of research for years to come.